
Most of the energy is carried off in the neutron. In a fusion power plant, the neutrons would heat a lithium blanket that, through a heat exchanger, would boil water for steam turbines in an electric power plant. In addition, reactions between the neutrons and the lithium nuclei, as described above, would breed more tritium.
The deuterium-tritium or D-T reaction is the most promising because of the forces between nuclear particles. The powerful mutual electrostatic repulsion of protons means that of all the elements, hydrogen nuclei offer the least repulsion and thereby require the lowest energy to get the reaction going. At very short distances, nuclear particles attract each other through the strong force, and the neutron in tritium adds to this attractive force, thereby promoting the fusion reaction. In this sense, a T-T reaction would seem better, but in fact it produces only 65% as much energy as D-T.
In the D-T reaction, each neutron carries off about 14 million electron volts of energy, roughly 80% of the released energy (an electron volt is the energy acquired by an electron in moving through a potential difference of one volt). These energetic neutrons constitute a considerable radiation hazard, so a fusion reactor will need a one-meter thick lithium blanket to absorb neutrons and breed more tritium. The strong neutron flux would necessitate robotic maintenance and control inside the blanket, and the radiation would make metal parts brittle. In addition, the danger of tritium leakage would require a steel container around the whole reactor.
For the deuterium and tritium nuclei to react, they must collide with very high energy. To some degree, quantum mechanics provides a way around the electrostatic repulsion of the protons, because it is possible for the two nuclei to “tunnel” through this barrier and thereby considerably reduce the necessary collision energy.
The easiest way to do D-T fusion is to accelerate deuterium nuclei into a high-velocity beam and aim it at a tritium target—tritium atoms locked in a solid. Such a machine operates as a research fusion reactor at the University of California, Berkeley. Thanks to the tunneling effect mentioned above, a 400, 000 electron-volt beam of deuterium ions produces 14 million electron volt electrons. The rate of energy release is about two watts.
For a power plant, though, a sustained reaction is necessary. To keep the fusion reaction going, the deuterium and tritium must be heated sufficiently that the ions’ thermal motion will produce sufficiently energetic collisions. In this case the ions must be hot enough that they will form a plasma. As a plasma moves, its electric currents produce electromagnetic forces that act back on the plasma, so controlling and confining the plasma is a daunting challenge.
As far as safety is concerned, a fusion power plant has significant advantages over a nuclear reactor. At any time, the fusion reactor contains enough fuel for only a few minutes operation, unlike the year’s worth of fuel in a fission reactor. If the plasma container is breached, the reaction stops immediately, so there is no danger of anything like a “China syndrome” meltdown. Also, there is no problem of long-term storage of spent fuel.
Research
Since the plasma is so hot, the ions must be kept off the walls of the container. One method is to confine the plasma with magnetic fields.
Magnetic Confinement:
Charged particles spiral around magnetic field lines, and if the container closes on itself, like a torus (a donut), the plasma can circulate within the container. Various approaches have been invented for shaping the magnetic fields needed to confine such a toroidal plasma, with different advantages and disadvantages.
Diagram of a coil would around a torus. Note that the wires are closer together on the inside of the torus and farther apart on the outside. When the wires carry electric current, the resulting magnetic field is therefore stronger in the inner part of the torus, so the plasma ions drift towards the walls.
A drawing of the ITER tokamak fusion reactor. The person shown in the foreground gives the scale of this device. Also, note that the torus cross-section is uniform—quite different from the stellarator. (published with permission of ITER)
Stellarator:
The stellarator, the first fusion machine, was designed by Lyman Spitzer in 1951. This device uses complicated coil windings, as shown in the illustration, outside the containment vessel to form the magnetic fields needed to confine the plasma. This approach avoids having to drive a large current in the plasma itself, but fabricating and positioning the magnets is a difficult challenge. The National Compact Stellarator Experiment (NCSX), a collaboration between the Oak Ridge National Laboratory (ORNL) and the Princeton Plasma Physics Laboratory (PPPL), is a proof-of-principle project to evaluate the viability of the compact stellarator. NCSX will cost about $74 million and be completed in 2007.
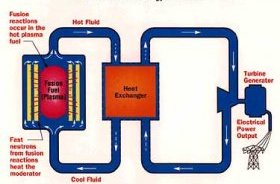

YOU MIGHT ALSO LIKE
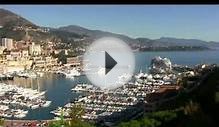
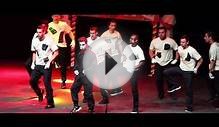
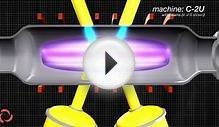