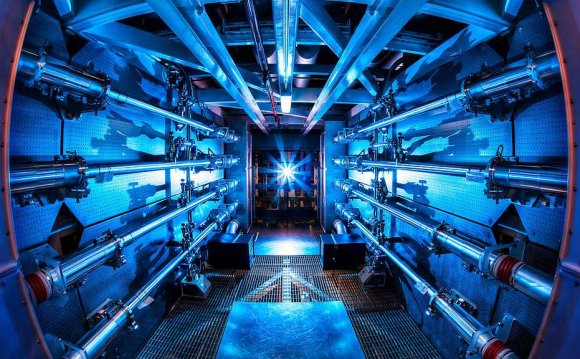
One of the biggest obstacles to making fusion power practical - and realizing its promise of virtually limitless and relatively clean energy - has been that computer models have been unable to predict how the hot, electrically charged gas inside a fusion reactor behaves under the intense heat and pressure required to make atoms stick together.
The key to making fusion work - that is, getting atoms of a heavy form of hydrogen called deuterium to stick together to form helium, releasing a huge amount of energy in the process - is to maintain a sufficiently high temperature and pressure to enable the atoms overcome their resistance to each other. But various kinds of turbulence can stir up this hot soup of particles and dissipate some of the intense heat, and a major problem has been to understand and predict exactly how this turbulence works, and thus how to overcome it.
A long-standing discrepancy between predictions and observed results in test reactors has been called "the great unsolved problem" in understanding the turbulence that leads to a loss of heat in fusion reactors. Solving this discrepancy is critical for predicting the performance of new fusion reactors such as the huge international collaborative project called ITER, under construction in France.
Now, researchers at MIT's Plasma Science and Fusion Center, in collaboration with others at the University of California at San Diego, General Atomics, and the Princeton Plasma Physics Laboratory, say that they have found the key. In a result so surprising that the researchers themselves found it hard to believe their own results at first, it turns out that interactions between turbulence at the tiniest scale, that of electrons, and turbulence at a scale 60 times larger, that of ions, can account for the mysterious mismatch between theory and experimental results.
The new findings are detailed in a pair of papers published in the journals Nuclear Fusion and AIP Physics of Plasmas, by MIT research scientist Nathan Howard, doctoral student Juan Ruiz Ruiz, Cecil and Ida Green Associate Professor in Engineering Anne White, and 12 collaborators.
"I'm extremely surprised" by the new results, White says. She adds that it took a thorough examination of the detailed results of computer simulations, along with matching experimental observations, to show that the counterintuitive result was real.
Persisting eddies
The expectation by physicists for more than a decade had been that turbulence associated with ions (atoms with an electric charge) was so much larger than turbulence caused by electrons - nearly two orders of magnitude smaller - that the latter would be completely smeared out by the much larger eddies. And even if the smaller eddies survived the larger-scale disruptions, the conventional thinking went, these electron-scale whirls would be so much smaller that their effects would be negligible.
The new findings show that this conventional wisdom was wrong on both counts. The two scales of turbulence do indeed coexist, the researchers found, and they interact with each other so strongly that it's impossible to understand their effects without including both kinds in any simulations.
However, it requires prodigious amounts of computer time to run simulations that encompass such widely disparate scales, explains Howard, who is the lead author on the paper detailing these simulations. Accomplishing each simulation required 15 million hours of computation, carried out by 17, 000 processors over a period of 37 days at the National Energy Research Scientific Computing Center - making this team the biggest user of that facility for the year. Using an ordinary MacBook Pro to run the full set of six simulations that the team carried out, Howard estimates, would have taken 3, 000 years.
YOU MIGHT ALSO LIKE


