
Extended Data Figure 1: The Borexino detector.
Hover over figure to zoom
The characteristic onion-like structure of the detector is displayed, with fluid volumes of increasing radiological purity towards the centre of the detector. Although solar neutrino measurements are made using events whose positions fall inside the innermost volume of scintillator (the fiducial volume, shown as spherical for illustrative purposes only), the large mass surrounding it is necessary to shield against environmental radioactivity. The water tank (17 m high) contains about 2, 100 t of ultraclean water. The diameter of the stainless steel sphere is 13.7 m, and that of the thin nylon inner vessel containing the scintillator is 8.5 m. The buffer and target scintillator masses are 889 and 278 t, respectively.
Extended Data Figure 2: Survival probability of electron-neutrinos produced by the different nuclear reactions in the Sun.
All the numbers are from Borexino (this paper for pp, ref. 17 for 7Be, ref. 18 for pep and ref. 19 for 8B with two different thresholds at 3 and 5 MeV). 7Be and pep neutrinos are mono-energetic. pp and 8B are emitted with a continuum of energy, and the reported (νe νe) value refers to the energy range contributing to the measurement. The violet band corresponds to the ±1 prediction of the MSW-LMA solution. It is calculated for the 8B solar neutrinos, considering their production region in the Sun which represents the other components well. The vertical error bars of each data point represent the ±1 interval; the horizontal uncertainty shows the neutrino energy range used in the measurement.
Extended Data Figure 3: The sequence of nuclear fusion reactions defining the pp chain in the Sun.
The pp neutrinos start the sequence 99.76% of the time.
Extended Data Figure 4: Study of the low energy part of the spectrum.
Comparison of the spectrum obtained with the main trigger (black) and by selecting events falling in the late part of the acquisition window triggered by preceding events (red). Above 45 struck PMTs, the spectral shapes coincide. The threshold effect for self-triggered events (black) is clear. The residual threshold effect at lower energy in the red curve is due to the finite efficiency for identifying very low-energy events within a triggered data window.
Extended Data Figure 5: 14C spectrum, and residuals, obtained from events triggered by a preceding event.

, Spectrum. , Relative residuals of a fit with the 14C β-emission spectrum (in units of standard deviations). The error bars thus represent ±1 intervals.
Extended Data Figure 6: Energy spectrum of the pile-up data for the standard cuts.
The small bump around 150 struck PMTs (~400 keV in Figs 2 and 3) is due to the pile-up of 14C with 210Po; at lower energies, pile-up is dominated by 14C+14C, and by 14C+dark noise.
Extended Data Figure 7: Energy distribution of events collected with no threshold applied.
The events correspond to regular, solicited triggers (sliced into 230 ns windows). This represents what the detector measures when randomly sampled. In an alternative treatment of pile-up, this spectrum is used to smear each spectral component used in the fit (see text for details).
Extended Data Figure 8: Distribution of best-fit values for the pp neutrino interaction rate.
Values are obtained by varying the fit conditions, including the fit energy range, synthetic-versus-analytic pile-up spectral shape, and energy estimator. The distribution shown is peaked around our reported value of 144 c.p.d. per 100 t.
Extended Data Figure 9: Goodness of fit versus pp neutrino interaction rate.


YOU MIGHT ALSO LIKE
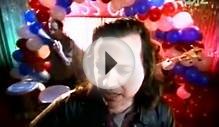
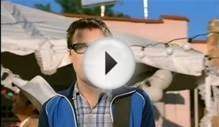
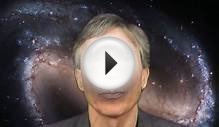